Battery Cells
Battery cells, commonly referred to as galvanic cells, harness electrical energy that is created due to differences in electrochemical potential between reactants and products in the cells. They contain the necessary components to store and release electrical energy for devices ranging from small electronics to vehicles and industrial systems.
The following annotated diagram depicts a typical rechargeable Li-ion battery cell. During the discharge process, lithium de-intercalates from the anode active material where it dissociates at the electrolyte into lithium cations (Li+) and an equal number of electrons. The Li+ ions in the electrolyte migrate through the separator and are inserted into the cathode active material. The electrons flow from the solid anode active material through the anode collector and leave the Li-ion battery cell as an electrical current. Charging is the reverse process.
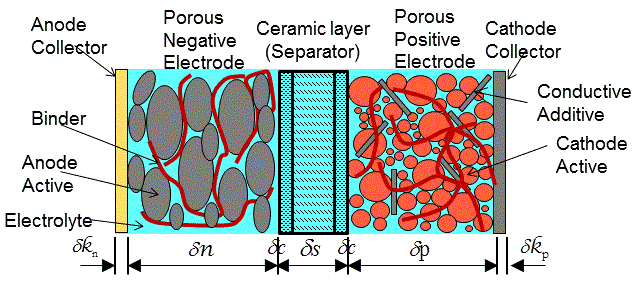
Disposable lithium batteries discharge in the same way, however, instead of using an intercalated lithium compound as the anode active material, a foil of metallic lithium is used—which is not typically recharged.
The electrical current that is created from Li-Ion battery cells can then power devices such as portable electronics, Hybrid Electric Vehicles (HEVs), and Plug-In Hybrid Electric Vehicles (PHEVs).
There are different types of battery cells, Simcenter STAR-CCM+ can be used to model lithium-ion and sodium cells.
Electrodes
Battery cells require both an anode and a cathode electrode to complete a circuit and allow current to flow. The electrodes allow the reversible insertion/extraction of ions into the active material phase (intercalation) for energy storage. This allows them to be closed systems, unlike fuel cells.
The essential properties of these electrodes are:
- Intercalation structure with vacant spaces that allow the insertion and extraction of lithium ions.
-
Conduction of electricity.
-
Occurrence of chemical reactions.
- Subgrid Particle Intercalation
- The Subgrid Particle Intercalation model resolves electrochemical reactions on geometrically unresolved surfaces by assuming that the active material is composed of spherically shaped particles into which ions can diffuse.
- 3D-MSE
- The ability to model the 3D micro-structure of an electrode and predict its electrochemical reactions, helps to determine the quality and performance of a cell design. For example, it is possible to determine how tortuous a porous electrode can be, and analyze or parametrize particle size and distribution, both key factors for an electrode. You can also determine the most effective setup to optimize high energy density–for mobile phones, high power output–for automotive applications, or a combination of both.
- Lumped
- If you are satisfied with a simplified representation of the irregular and complex structure of the active material, for each electrode you can create a simple solid structure of randomly distributed solid particles that form one part.
- Combination
- If you are only concerned with the detailed reactions which occur at one of the electrodes—for example, sacrificial anode depletion, you can create a detailed 3D-MSE representation for the electrode of interest, then create a lumped electrode to represent the other electrode.
- Disposable
- If you are modeling a disposable battery, you can create the cathode using the lumped or 3D-MSE approach. Then, for the anode, you create a thin solid part to represent a foil of lithium metal.
Electrolytes
An electrolyte is a combination of lithium/salt and solvent. When an electrochemical potential is applied, the electrolyte transports charge through movement of ions from the negative current collector to the opposing positive current collector. This process is also referred to as salt migration.
You can use the Concentrated Electrolyte model (for multi-component liquids) or the Li-Ion Concentration model (for liquids) to account for the transport of lithium ions in the electrolyte. These models are derived from the Doyle-Fuller-Newman transport model ([854]), which models the behavior of solutions containing a high concentration of ions that are typically referred to as concentrated electrolytes.
Simcenter STAR-CCM+ assumes an electroneutral electrolyte—consisting of a solvent that embeds a binary salt that decomposes into a cation and an anion. As a consequence, only a single lithium/salt concentration scalar is solved for, which is understood to be the concentration of either the salt, the cation, or the anion.
For concentrated electrolyte solutions, the interactions between ions become significant, and their behavior can deviate from that predicted by ideal solutions or dilute electrolyte models. Non-ideal electrolyte properties, including the diffusion coefficients, are important factors in modeling electrolytes. To account for these properties, the concentrated electrolyte model allows for concentration and temperature dependent diffusion coefficients.
- Li-Ion Battery Cell model—uses the Li-Ion Concentration model to model the concentration of salt in electrolytes, as well as the concentration of lithium in active parts of an electrode. The Li-Ion Electric Potential model determines the electric potential in solid and electrolyte regions. See (Modeling Li-Ion Battery Cells).
- Simcenter STAR-CCM+ 3D Cell Design Capability—is a template that contains pre-configured setups for simplified and accelerated battery simulations in Simcenter STAR-CCM+. The template uses the Concentrated Electrolyte model for the electrolyte transport and the Subgrid Particle Intercalation model for the storage of ions in the electrodes. The Concentrated Electrolyte model requires the Electrodynamic Potential model to determine the electric potential and current in the electrolyte regions.
Both methods use liquid electrolytes to simulate lithium-ion batteries and account for the movement of charged particles in porous electrodes, intercalation reactions within the electrodes, charge transfer reactions, internal particle diffusion, and the influence of temperature on transport properties.